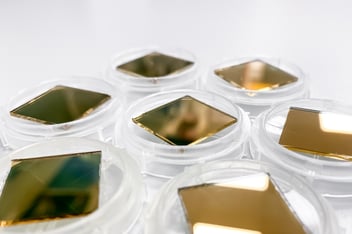
What are the best nanomaterials for biosensors?
In order to choose the best nanomaterial for specific types of biosensors, engineers need to understand the features, benefits and challenges of each.
Drug discovery remains one of the most resource-intensive undertakings in the pharmaceutical industry, requiring over a decade and potentially millions in investment to bring a single therapeutic to market.
Across this process, from early screening to clinical trials, precision, speed and reliability are extremely important. Yet conventional technologies frequently fall short, hampered by throughput limitations, complex sample preparation and inadequate sensitivity.
Nanomaterial-based biosensors, particularly those built on carbon-based platforms, can change how companies navigate this complexity. Let’s take a look at the critical stages of drug discovery, the persistent challenges they present and how nanomaterial-enabled biosensing can offer transformative solutions.
The discovery journey begins with high-throughput screening (HTS), where vast libraries of compounds are evaluated for activity against a given biological target. Traditional HTS platforms are often reliant on fluorescent labels or radioisotopes, which are labour-intensive, require significant reagent volumes and lack real-time feedback.
Nanomaterial-based electrochemical biosensors, especially those using high surface area carbon-based materials, dramatically improve signal-to-noise ratios for molecular binding events. This leads to label-free, real-time screening with femtomolar sensitivity as well as multiplexed configurations for the parallel assessment of multiple targets, improving both throughput and specificity in early discovery phases.
Following the initial hit identification, the lead optimisation phase involves testing and modifying compounds to improve their binding affinity, stability and specificity. Here, a detailed understanding of the drug-target interaction is critical. Traditional techniques like surface plasmon resonance or calorimetry, whilst precise, are cumbersome and expensive. They often require purified components and idealised conditions, far from the complexity of physiological environments.
Carbon-based electrochemical biosensors provide a label-free and physiologically relevant way to probe molecular interactions. This allows researchers to study kinetics and affinity directly in serum, saliva or other complex fluids in a cost effective and efficient manner. Such capabilities are invaluable when characterising leads that target extracellular proteins, enzymes or metabolic pathways.As drug development progresses toward the clinic, identifying which patients will benefit from a drug becomes a central concern. Companion diagnostics are essential tools in stratified medicine, but conventional platforms often require lab infrastructure and slow turnaround times. Current diagnostic workflows rely on ELISA, PCR or mass spectrometry, which require centralised labs, trained personnel and long sample-to-result intervals. They are not well-suited for real-time or point-of-care use.
Nanomaterial-based biosensors excel in the companion diagnostics domain because they operate directly in biofluids with minimal preparation. For example, new carbon-based electrodes have been successfully deployed to detect biomarkers like interleukin-10 and salivary cortisol with ultra-low detection limits and high specificity. The result is portable, rapid-response devices capable of performing companion diagnostics at a fraction of the traditional cost.
Even the best technologies on paper need to ultimately prove themselves in clinical workflows. For biosensors, this means ensuring performance in real-world settings, across temperature variations, biological matrices and patient-to-patient variability. Sensor drift, surface fouling and cross-reactivity can all compromise the reliability of biosensor platforms. Additionally, reproducibility and scale-up remain key concerns for regulatory approval.
New carbon-based nanomaterials show significant advantages in mechanical durability and anti-fouling performance. Their structure allows analyte access while maintaining high electrical contact. Studies using new carbon-based electrodes have demonstrated stability across repeated measurements and minimal signal loss in artificial sweat, saliva and serum. As the field advances, new anti-fouling coatings, modular sensor architectures, and plug-and-play microfluidics are accelerating the move toward clinically viable, scalable biosensor platforms.
From high-throughput screening to companion diagnostics, carbon-based electrochemical biosensors are addressing some of the most persistent challenges in drug discovery. They deliver real-time, label-free, ultra-sensitive detection in complex biological environments. However, traditional carbon-based nanomaterials like graphene are difficult to produce, expensive and suffer from issues like biofouling.
At the centre of this shift is Gii, a next-generation material engineered for biosensing. With unmatched conductivity, functional versatility and proven performance in physiological fluids, Gii is redefining what's possible for developers of diagnostics, therapeutics, and point-of-care technologies.
In order to choose the best nanomaterial for specific types of biosensors, engineers need to understand the features, benefits and challenges of each.
We go into detail surrounding biosensors, unpacking their components, use cases and application examples in different industries.
We examine the strengths of carbon nanostructures and their impact on biosensor innovation, as well as their weaknesses and how to overcome them.